Build A Organelle in Three Weeks, and Get Published in Cell
From an iGEM project to a Cell paper. A true story behind the first synthetic membraneless organelle in bacteria.
Fantastic Organelles and How to Build Them (Chapter 3), also on the Ailurus website
Imagine starting a new field in weeks, building a synthetic organelle from scratch
Today, I'd like to share the true story behind the first synthetic membraneless organelle in bacteria. It was also likely the first liquid-liquid phase separation (LLPS) system ever reported in bacteria.
Although it was eventually published in the journal Cell, years after its first public record in 2017, the original work was actually created in just three hectic weeks.
It All Started with an iGEM Team...
And a young, fresh PhD student (yes, that's me) took the job of mentorship at the last minute.
Since 2004, iGEM has promoted synthetic biology through student-led competition. In the early days, many important research discoveries were first reported at iGEM before publishing in peer-reviewed journals, including the first synthetic DNA scaffold, and even some of the earliest AI-synthetic biology integrations.
The lab I was in, and its education arm, the Center for Research and Interdisciplinarity (CRI), hosted the first iGEM team in France, iGEM Paris Bettencourt, since 2007. There was a strong mentoring system that helped the team consistently earn awards, make it to the finals, and even win a grand prize in 2013, until...
In 2017, old-time mentors decided to take a break for the 10-year anniversary. Meanwhile, a group of Master’s students at CRI still insisted on competing in iGEM that year, and started to organize events all by themselves anyway.
Oh, drama. I liked it.
Out of curiosity, I attended one of their meetings, only to find them struggling with project ideas for over an hour, unable to make any progress. Apparently, in the absence of mentors, they were not making much progress. It was already the end of April. They were facing the deadline for registering as a team and all the payments. They needed support, or they wouldn't be able to move forward.
Here came the conversation with the big boss — Ariel. Ariel, a leading expert in synthetic biology, cofounded CRI and has mentored the iGEM Paris team since its inception.
It was April 27th, a typical Paris Thursday day, rainy and chilly. Here in a cozy café, a few tables, people gathered, arguing, and looking for a solution.
"Why are you here?", Ariel turned to me and asked.
"Emmm...", honestly, I just wanted to grab a beer after work. "I don’t know?" I replied.
"Good. You should mentor them to do iGEM."
"Huh? Okay?"
Somehow, I was convinced to become the team's main instructor, to bring structure and guide the team through the process. With my colleagues joining the mentor team, things were finally kicked off.
"We Need a Project"
But there was still a major problem: there was no project!
Let me elaborate how scary it was. iGEM is a competition with hard deadlines in October, meaning we had only five months to complete lab training, conduct experiments, create models, perform outreach, and more. Not to mention, summer vacations in Paris would make it even harder to progress.
Of course, for the purpose of education, we prefer to have students come up with their own ideas, but by 2017, it was tough to find something truly novel in synthetic biology. Over a thousand teams participated in iGEM. Proposing an idea that hadn't been tried before was challenging, even for experienced scientists.
While struggling to mentor them to come up with their "own" idea, I decided to prepare something "safer" - something simple enough to accomplish with our lab's resources, yet novel enough that no one had done it before.
And the best approach was to combine well-established components in a completely new way.
Connecting the Dots: From RNA Phase Transition
On June 6, a new Nature paper arrived and saved my day. It showed that expanded triplet repeats of RNA, like the long "CAGCAGCAG..." sequences associated with Huntington's diseases, form liquid droplets in human cells. Ariel, knowing my background in RNA synthetic biology, forwarded it to me. All of a sudden, there seemed to be an obvious project.
RNA triplets undergo phase separation in human cells, but apparently no one had the time to try it in bacteria. Novelty? Check!
Our lab had the necessary tools for RNA engineering since our Science publication in 2011, including a split green fluroescent protein (GFP) system to visualize RNA scaffolds. Also, we have years of experience using fluorescence microscopy to study subcellular structures in bacteria, and thus were well-prepared to observe LLPS behavior if it occurred.
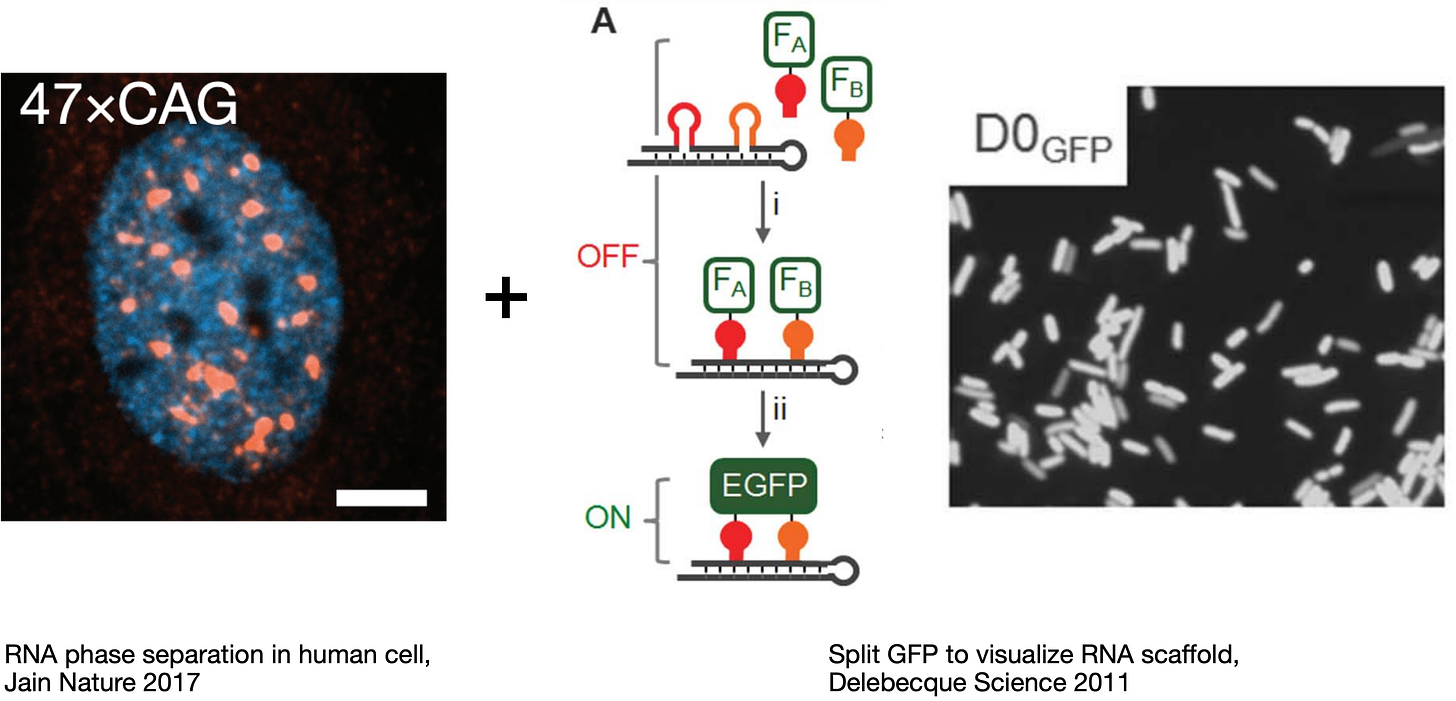
We just needed the RNA repeats. I happened to know the group behind the Nature paper, and quickly contacted Ankur and Ron for the materials. We finally obtained the key plasmids by September. Ariel also found some Huntington-related plasmids from an old project decades ago.
"Hurry! We Have to Finish This!"
One of the team members, Joe, took charge of the project. By August and September, Joe was comfortable with everything in the lab, and finally, by October 1st, we had all the genetic materials ready.
30 days before the deadline. Greeeaaat! Let's get started.
In the normal way of research, we probably would start to label the RNAs with full GFP to observe whether they form structures. However, since we had no time for trial and error at all, I decided to bet on a non-trivial system.
It involved this split GFP system, cutting GFP into two inactive halves FA and FB. Without any scaffolding, FA and FB spontaneously form active GFP at a relatively low level. And RNA is designed to undergo phase separation and recruit FA parts. We counted on FA to sequentially recruit FB, and light up fluorescence foci in E. coli.
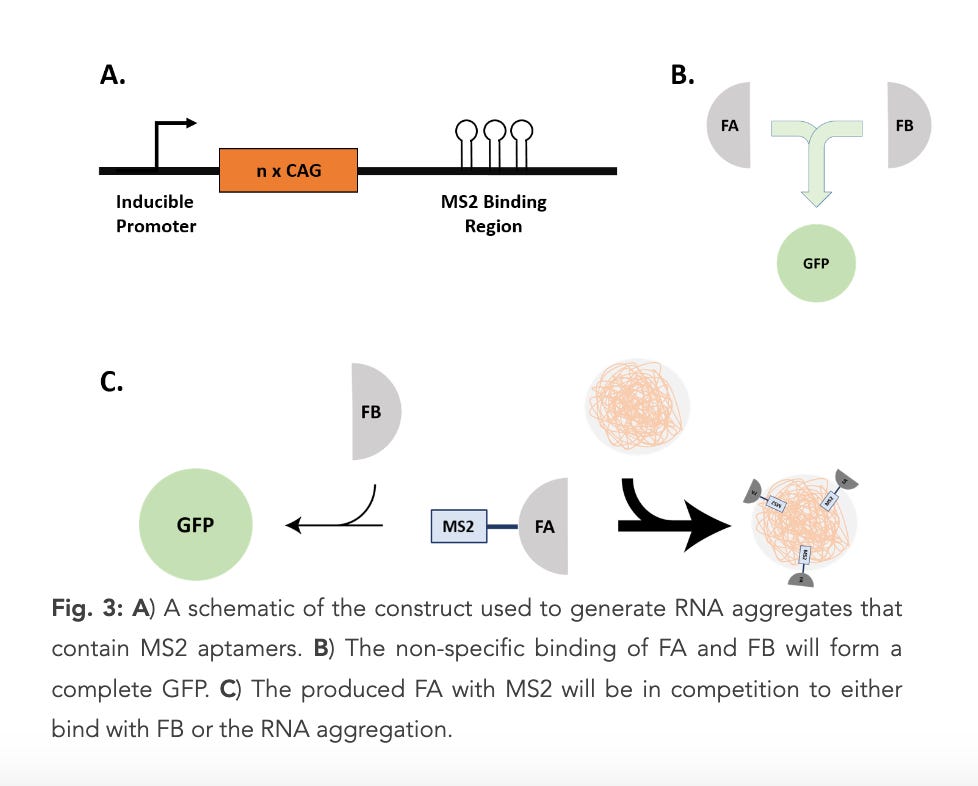
If it worked, it would be a strong enough case to show both the existence of physical structure, and its functionality to enhance biochemical reactions. If any of these steps failed, we would be so doomed.
By October 18th, we finished cloning the plasmids, transformed them into E. coli, and were finally able to collect data.
Only 13 Days LEFT! Yeaaah....
The very next day, Ana, who was helping Joe with the microscope, came up to me and said, "What are you guys working on? It looks beautiful, like stars." Apparently, we weren’t screwed - it was working!
In a repeated experiment, I saw the image. "WOW!" It was so remarkable that I immediately took a photo from the computer screen, and shared it with the team.
These multiple micron-scale "RNA organelles" could coexist in a single cell, far larger than any synthetic scaffolds we'd previously made. We also observed two-layer structures inside many droplets, later proven to be secondary phase separation - a hallmark of liquid properties.
"Let's fully characterize its properties." Joe and I worked some extra nights together, pipeting cells into different culture conditions, and visualize the system at various time points.
The system rewarded us with its remarkably complex behaviors that matched a simplified biophysical model I wrote. During exponential growth, the FA-FB binding remained at the same level, regardless of how much protein was expressed, demonstrating a behavior of buffering and robustness. In the stationary phase, it dramatically increased, forming bright fluorescent foci, as a catalyst of the reaction.
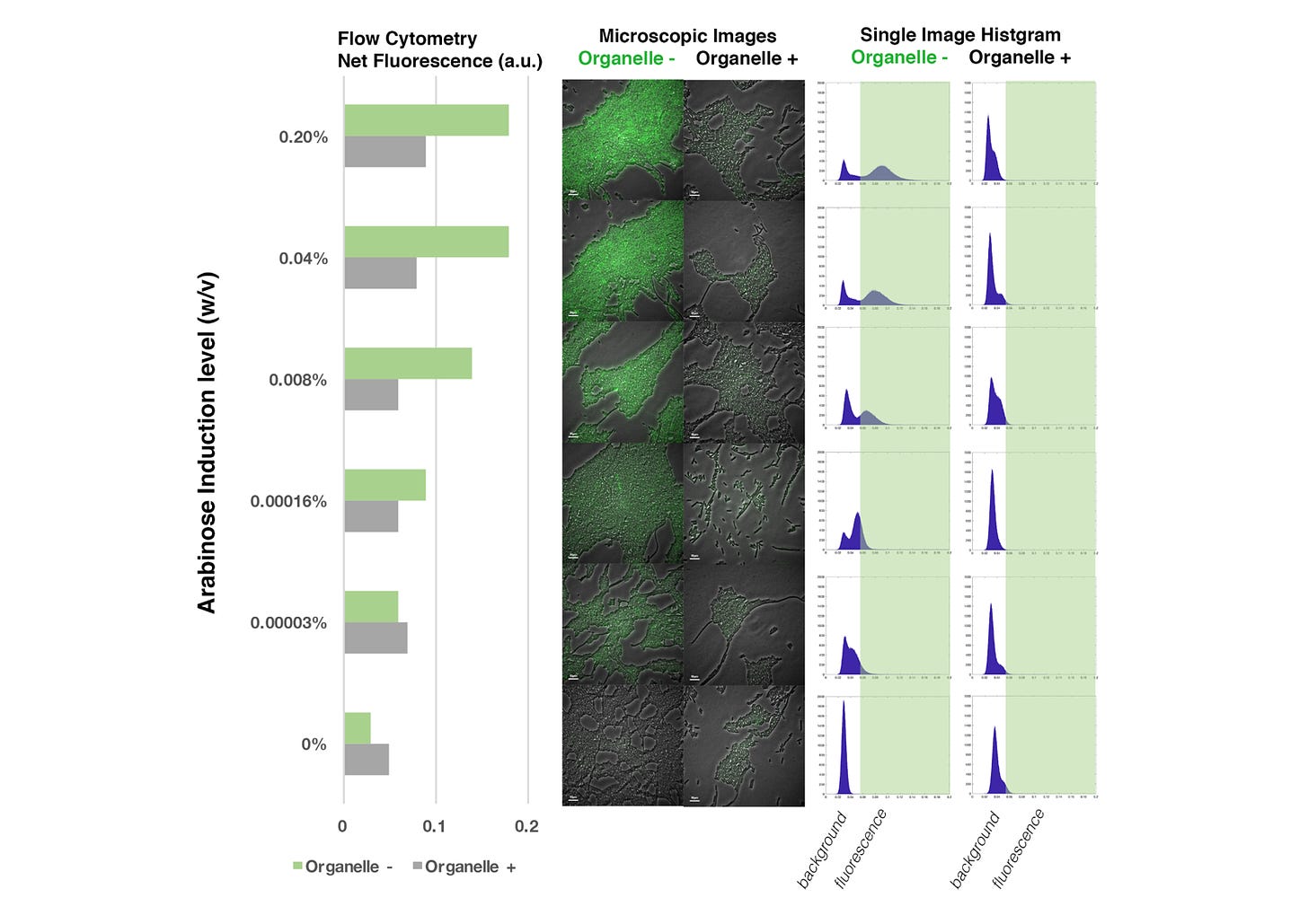
As far as I know, this was the first example of robustness tuning and non-equilibrium tuning in a phase separation system.
iGEM Competition and After iGEM
Incredibly, we completed everything, from cloning the plasmids to characterizing the system, in just three weeks!
The results were published on the iGEM wiki, and we presented them at the Jamboree.
After iGEM, Joe, myself, and other colleagues continued working on the system. Ariel and I eventually wrapped and published some of the results in Cell by 2022, five years after we first reported it - a story to tell another time. Nevertheless, what happened in those three weeks not only built the core of this article, but also contributed a majority of published data.
First LLPS Reported in Bacteria?!
Thanks to my outsider ignorance, I did not realize the challenges. Adapting Richard Feynman's quote, "What I cannot create, I do not understand," what I do not understand, I created it anyway.
Looking back, it was a huge, risky leap.
Our work during iGEM 2017 was probably the first report of LLPS in bacteria. Until 2018, natural phase separation had only been reported in eukaryotic cells. It was unclear whether LLPS was even possible in bacteria, let alone engineering it.
Constructing artificial LLPS in eukaryotic cells also had just begun in 2017. There were significant unknowns, even today. Like this newly reported RNA phase transition, would it actually require some unknown protein component in human cells, who knows?
The Power of Modular Design
We definitely had beginner's luck, but part of our success was due to good design: the application of modular design. This is a strategy often used in synthetic biology, to break a complex system into smaller modules that can be created, engineered or replaced independently.
In our case, RNA molecules only build the structure, to recruit other components that provided the actual functionality. In this way, we could design many RNA molecules and function components, test them separately, and assemble a new system by combination. It also allowed us to compound the knowledge and reuse historical components, RNA repeats from Nature paper, split GFP from Science paper 10 years ago, and later a GFP plasmid even 15 years go.
Outside synthetic biology, it's still common to directly link phase separation to functional domain. Then, every time you study a new system, you not only need to clone new encoding DNAs, but also have to face the potential instability and undesirable features of new molecules.
Summary of Design Principles
How to Build a Synthetic Membraneless Organelle? Our practice demonstrates a good framework:
Express a molecule as the building block: It should contain phase-separating domains and recruitment domains.
Express other molecules to add functionality: These molecules should have functional domains and tags that target the recruitment domains.
This framework lays the foundation for more sophisticated designs.
There are many attempts to construct synthetic membraneless organelles after our work in 2017. Some of them worked, and some of them did not. From all these results, we could further understand the rules underlying engineering these phase separation systems - a story for the next time.
Notes: This RNA organelle project cannot be achieved without team members and mentors from iGEM Paris Bettencourt team 2017, especially Joseph Ryan & Ariel B. Lindner in this story, as well as the supports from Ana Santos, Antoine Decrulle, Chantal Lotton, Xiaohu Song from INSERM U1001, and Xiaowei Yan, Ankur Jain & Ron Vale from Vale lab. Thanks to colleagues from Ailurus for reading drafts of this.
Haotian Guo is the founder and CEO of Ailurus Bio.
Ailurus is a pioneering biocomputer company programming biology as living smart devices, with products like PandaPure® that streamline protein expression and purification directly within cells, eliminating the need for columns or beads. Our mission is to make biology a general-purpose technology - easy to use and as accessible as modern computers. See more at https://www.ailurus.bio
More at Fantastic Organelles and How to Build Them
This is very similar to what I was thinking in 2001, but I had no proof, and like you said, research was lacking. It is amazing to see your group tackle this and come to very conclusive evidence!!